Microbial cells can exhibit different growth patterns depending on the availability of growth nutrients in their immediate environment. In batch culture, microbial cells cannot grow continuously because the growth nutrients required for development is not renewed after it has been depleted. Thus exponential growth is limited to a few generations of microbial cells in batch cultures because the system is a closed system of microbial cultivation. Microorganisms cultivated in test tubes are typical examples of a batch or closed system of growth.
Only continuous culture can support the exponential growth of bacterial cells over a long period of time because such a system of microbial culture is an open system which is flexible and allows for nutrient replacement after their depletion. Continuous culture system is a growth method that maintains a constant environmental growth conditions for microbial cells through the continual provision of nutrients and removal of toxic wastes from the growth medium. It is an open system of growth that maintains microbial population in the log or exponential phase; and one that maintains growth for extensive periods of time. Continuous culture is usually carried out in a specialized system known as chemostat (Figure 1).
In a chemostat, fresh nutrient is supplied to meet up with the microbial growth. Excess microbial cells (i.e. spent cells) and toxic wastes are immediately removed from the culture medium as soon as they develop. The two methods used for carrying out a continuous culture system of microbial growth are chemostats and turbidostats. Turbidostats is an open system method of continuous culture in which nutrients are constantly provided and toxic wastes removed as they are formed (as obtainable in the chemostats). It is so named because it evaluates the turbidity of bacterial culture in a growth vessel during the log phase of growth.
Turbidity is the measurement of suspended solids (in this case, microbial cells) in a solvent e.g. water or normal saline. The turbidostats is similar to the chemostats except that the former functions at a higher dilution rate while the later runs at a lower dilution rate.
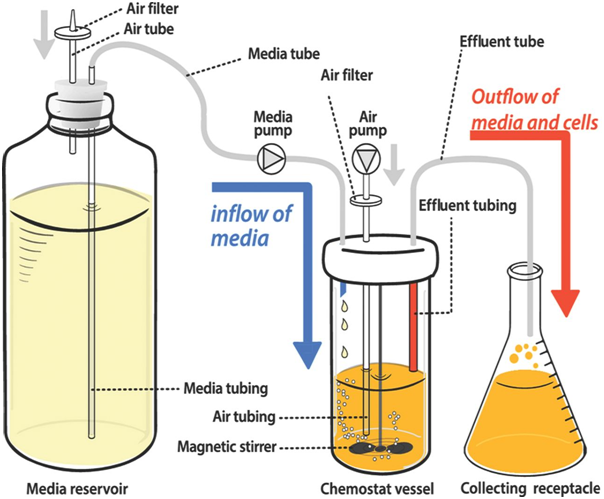
A chemostat is simply a static chemical and/or biological environment in which a controlled chemical/biological reaction takes place. It is a bioreactor to which fresh medium for the growth of an organism is continuously added. It is however noteworthy that as the growth medium is continuously introduced into the vessel; the culture liquid generated in the process is also continuously removed from the bioreactor in order to keep the culture volume of the vessel constant and operating at an optimal condition.
By changing the rate with which fresh growth medium is added to the bioreactor (i.e. the chemostat) the growth rate of the microorganism being cultivated can be easily controlled in real time. A chemostat has fittings for effluents generated during the process; and portals for influents, agitation of the medium and source for the introduction of filtered air are also made available in it. With the chemostat, scientists can identify and better understand how cells especially microorganisms and other forms of life regulate their growth; and also to understand the adaptive evolution of living organisms. Chemostat enables precise control of the selective pressure under which organisms evolve and it facilitates experimental control of cell growth rate.
PHASES OF MICROBIAL GROWTH
LAG PHASE
Lag phase represent the stage in the development of a bacterial cell when no growth occurs. It is the period after the initial seeding, culturing or inoculation of a bacterium in a fresh culture/growth medium before observable growth begins (Figure 2). When bacterial cells are initially introduced into a new medium or environment, they usually require some time to synthesize important biosynthetic intermediates (i.e. primary metabolites) required to keep them alive. Microbial cells initially adjust to new medium until they can conveniently divide frequently by binary fission (as exemplified by the log phase). During lag phase, the bacterial cell monitors their new environment and become acclimatized to it. Lag phase represents a period in the cell division of bacteria in which the respective bacterial cells are maturing or growing but on the other hand, they are not able to divide and increase in their population size. There is enormous synthesis of primary metabolites such as enzymes, nucleic acids and alcohol which are critical to the growth of the bacterial cell.
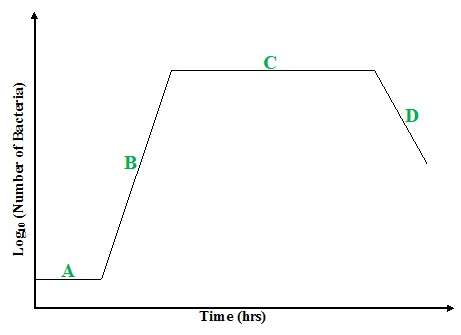
Keys:
A = Lag phase
B = Log (exponential Phase)
C = Stationary phase
D = Decline & Death Phase
LOG PHASE
Log phase is the period in the growth curve of a microorganism in which the growth of the bacteria increases at an exponential time. This stage of microbial growth is also known as exponential growth phase; and it is characterized by doubling of the bacterial cells (Figure 2). In this stage, the number of new bacteria that appears per unit time is equal to the present population of bacteria. The doubling of the bacterial cells continues at a constant rate unless the growth is inhibited by altering some of the growth conditions. Therefore, the number of bacterial cells and the rate of population increase doubles at each consecutive time period. When the natural logarithm of bacterial cell number is plotted against time (hrs) for this type of exponential growth, a straight line graph is obtained. The slope of this graph (line) gives the specific growth rate of the bacteria. The specific growth rate is a measure of the number of divisions per cell of the bacteria per unit time. Therefore the slope of the line or graph represents the actual rate of the cell growth; and this is usually dependent on the prevailing growth conditions in the medium. This growth conditions may affect the frequency of cell division events in the growth medium, and thus the survivability of the resulting daughter cells. The growth and doubling of the bacterial cells at the log or exponential phase of growth can be inhibited following nutrient depletion and the buildup of toxic wastes. Studies of microbial metabolism and physiology are usually undertaken at the exponential growth phase because the microbial cells are often at their prime or maximum growth in this stage.
STATIONARY PHASE
Stationary phase is the period in the growth curve of microorganisms (bacteria) in which the growth of the microbial cells stops. The stationary phase is characterized by a slow growth of bacterial cells due to nutrient depletion and toxic waste buildup in the growth medium. Stationary phase sets in as the growing bacterial cells begin to use up or exhaust all the growth nutrients available to them. Once the stationary phase sets in, the line of the growth curve flattens and assumes a horizontal dimension (Figure 2) because the rate of bacterial growth is equal to the rate of bacteria death. Growth rate is stabilized at this phase as the cell number of the organism is no longer increasing. However, the stationary phase is important because it is at this phase that secondary metabolites (e.g. antibiotics) with immense industrial and economic values are synthesized.
DECLINE AND DEATH PHASE
The growth of a bacterial cell begins to decline and recede once growth nutrients become unavailable and drastically depleted in the growth medium. In some circumstances, the microbial cells may continue to grow and metabolize but such growth is not enduring because the microbial population will eventually die (Figure 2). The decline stage ushers in the death phase which is generally characterized by a period of “no growth”. At the death stage, the rate of bacterial death exceeds the rate of bacterial growth. Growth stops entirely because nutrient has been depleted and toxic wastes have started building up. Bacteria completely loses its ability to reproduce (i.e. grow & divide) at this stage of the growth cycle. However, bacterial cells capable of forming endospores and other protective factors that helps it to survive adverse environmental conditions can survive this stage of the growth cycle until it finds a suitable environment to continue its growth process.
References
Brooks G.F., Butel J.S and Morse S.A (2004). Medical Microbiology, 23rd edition. McGraw Hill Publishers. USA. Pp. 248-260.
Madigan M.T., Martinko J.M., Dunlap P.V and Clark D.P (2009). Brock Biology of microorganisms. 12th edition. Pearson Benjamin Cummings Publishers. USA. Pp.795-796.
Prescott L.M., Harley J.P and Klein D.A (2005). Microbiology. 6th ed. McGraw Hill Publishers, USA. Pp. 296-299.
Ryan K, Ray C.G, Ahmed N, Drew W.L and Plorde J (2010). Sherris Medical Microbiology. Fifth edition. McGraw-Hill Publishers, USA.
Singleton P and Sainsbury D (1995). Dictionary of microbiology and molecular biology, 3rd ed. New York: John Wiley and Sons.
Talaro, Kathleen P (2005). Foundations in Microbiology. 5th edition. McGraw-Hill Companies Inc., New York, USA.
Discover more from #1 Microbiology Resource Hub
Subscribe to get the latest posts to your email.